Editorial
Edible vaccines to combat Infectious Bursal Disease of poultry
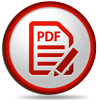
Muhammad Sarwar Khan*
Center of Agricultural Biochemistry and Biotechnology (CABB), University of Agriculture, University Road, P. O. Box 38040, Faisalabad, Pakistan
*Address for Correspondence: Muhammad Sarwar Khan, Center of Agricultural Biochemistry and Biotechnology (CABB), University of Agriculture, University Road, P. O. Box 38040, Faisalabad, Pakistan, E-mail: sarwarkhan_40@hotmail.com
Dates: Submitted: 30 October 2018; Approved: 08 November 2018; Published: 09 November 2018
How to cite this article: Khan MS. Edible vaccines to combat Infectious Bursal Disease of poultry. Arch Biotechnol Biomed. 2018; 2: 018-021. DOI: 10.29328/journal.abb.1001012
Copyright License: © 2018 Khan MS. This is an open access article distributed under the Creative Commons Attribution License, which permits unrestricted use, distribution, and reproduction in any medium, provided the original work is properly cited.
Poultry industry is a domineering section of agriculture sector in the world as it provides meat, income and employment. Of the poultry industry, broiler chicken is dominating, as US export was more than 41 billion pounds of chicken (about 16.5 percent of production) in 2017 [1]. In Pakistan, the poultry industry contributes around 1.4 percent to the GDP and 31 percent to total meat production [2]. The global demand for this meat is rising in developing world including Pakistan. To meet the needs, rearing of poultry at both domestic and commercial levels is imperative [3]. However, the industry faces a lot of constraints, preventing it from reaching its maximal potential. Poor welfare, insufficient quality nutrition and devastating diseases are some of these problems. Amongst the myriad of diseases, Infectious Bursal Disease (also known as Gumboro disease, infectious avian nephrosis and infectious bursitis) is an acute, contagious viral disease of chicken [4-6]. It not only affects domesticated but also wild poultry throughout the world by targeting the immune system. [7-9].
Gumboro disease is caused by Infectious Bursal Disease Virus (IBDV), which is a member of genus Avibirnavirus of the Birnaviridae family. IBDV is a non-enveloped virus having a capsid that contains a double stranded RNA genome, split into two segments A and B [10]. Segment A of the genome encodes four proteins (VP2, VP3, VP4 and VP5) while segment B encodes VP1. Based on Pathogenicity the IBDV strains can be grouped into three pathotypes; classical virulent, antigenic variant and very virulent [11]. Though the first outbreak of very virulent IBD virus was reported in Europe in early 1990s [12], recent pandemics across Asia, Africa and South America [13] have heavily damaged commercial and wild poultry. Contamination of a rearing site with the IBDV leads to a horizontal transmission of disease across the flocks through contaminated feed and water. However, vertical transmission is not reported yet [14]. Classical virulent strains flaunt a mortality rate as high as 20-30%, mostly due to the widespread bursal damage in infected poultry [15]. Classical virulent strains are the source of commercially available vaccines against IBD viral infections. In early 1980s, new antigenic variants emerged, causing more than 50 percent mortality in birds by rapid bursal damage [16]. Current vaccines prepared from classical strains have failed to control the disease caused by these antigenically variant strains. In the late 1980s, very virulent IBD virus strains were reported that induced IBD with a more pronounced bursal lesion and higher mortality (up to 90 percent) when compared to other IBDV strains [17]. In addition, vaccination against the very virulent strains encounters many challenges as very virulent IBD virus can breakthrough protective antibodies, and therefore requires more efficient vaccination approaches.
Presently, killed and attenuated live viruses are used as vaccines to immunize poultry against infectious diseases. Despite all efforts, consistent outbreaks, even in vaccinated flocks are a source of concern. The probable reasons may include poor biosecurity measures and unrepressed viral shedding (release of virus particles from an infected host cell following successful reproduction). Vaccines prevent viral shedding more proficiently if these are developed from strains homologous to currently circulating genotypes [18], this warrants molecular characterization of evolving IBDV strains.
One of the strategies to develop cost-effective and clean vaccines is expressing the engineered antigenic genes in edible plants. Such genes may be expressed from nuclear genome, both to accumulate the protein in the cytoplasm or targeting to plastids, expressing engineered genes from the chloroplast genome [19,20]. Of these strategies, expressing genes from chloroplast genome is more advantageous since proteins may accumulate to exceptionally high levels with bona fide structures [21,22]. High level expression in chloroplast is due to the polyploid nature of the plastid type and plastome (the plastid genome). Further, presence of chaperonin proteins in plastids [23] helps proteins to be biologically active. Precisely describing the technology, a gene of interest under the control of a promoter and a terminator along with another gene that confers resistance to an antibiotic is cloned between the DNA fragments from the plastome - used as left and right borders - to target the enclaved genes into the plastome through two homologous recombination events [24,25]. The antibiotic gene is used to select and screen the recipient plants. Poultry vaccines (edible or non-edible, Figure 1) have been developed successfully from plants where nuclear genome is transformed [26,27], however, from plants with transformed chloroplasts are underway. Leaves of plants with uniformly transformed genome (developed using gene gun or Agrobacterium-mediated method of transformation) are used either to extract the expressed antigenic proteins (in case of non-edible plants) or to directly feed birds (in case of edible plants). However, seeds are used in the feed or to store the transproteins longer period without the cold-chain requirements. Vaccines developed by expressing vp2 gene of IBD have induced Ig G response and immune protection in one to four-weeks-old chicks [26]. When vp2 gene was expressed in rice and grains were used to immunize the chicks, neutralizing antibodies were produced that protected birds from very virulent strains when double amount of antigenic protein was orally administered [27].
Figure 1: Transgenic plants expressing vp2 gene. A. Edible transgenic rice plant carrying VP2 antigenic protein in seeds. B. Non-edible transgenic tobacco plant carrying VP2 antigenic protein in leaves.
References
- Farhanah MI, Yasmin AR, Isa NM, Hair-Bejo M, Ideris A, et al. Bursal transcriptome profiling of different inbred chicken lines reveals key differentially expressed genes at 3 days postinfection with very virulent infectious bursal disease virus. Journal of General Virology 2018; 99: 21–35. Ref.: https://goo.gl/odUMze
- Economic survey of Pakistan. 2016-17. Ref.: https://goo.gl/A189mb
- Khan MS, Khan IA. Biopharming: A Biosecurity Measure to Combat Newcastle Disease for Household Food Security. Biosafety 2015; 4: e156. Ref.: https://goo.gl/SG8rgw
- Shabbir MZ, Zohari S, Yaqub T, Nazir J, Shabbir MA, et al. Genetic diversity of Newcastle disease virus in Pakistan: a countrywide perspective. Virol J. 2013; 10: 170. Ref.: https://goo.gl/cMd9zo
- Müller H, Mundt E, Eterradossi N, Islam MR. Current status of vaccines against infectious bursal disease. Avian Pathol. 2012; 41: 133-139. Ref.: https://goo.gl/S4uPwc
- Mogensen TH. Pathogen recognition and inflammatory signaling in innate immune defenses. Clin Microbial Rev. 2009; 22: 240-273. Ref.: https://goo.gl/A4sp3a
- Rehman ZU, Meng C, Umar S, Munir M, Ding C. Interaction of infectious bursal disease virus with the immune system of poultry. World's Poultry Sci J. 2016; 72: 805-820. Ref.: https://goo.gl/m9Rgby
- Mundt E. Tissue culture infectivity of different strains of infectious bursal disease virus is determined by distinct amino acids in VP2. J Gen Virol. 1999; 80: 2067-2076. Ref.: https://goo.gl/Xg81XD
- Nielsen O. Sørensen P, Hedemand J, Laursen S, Jørgensen PH. Inflammatory response of different chicken lines and B haplotypes to infection with infectious bursal disease virus. Avian Pathol. 1998; 27: 181-189. Ref.: https://goo.gl/BDEhL5
- Muller H, Scholtissek C, Becht H. The genome of infectious bursal disease virus consists of two segments of double-stranded RNA. J Virol. 1979; 31: 584-589. Ref.:
- Ture O, Saif Y, Jackwood D. Restriction fragment length polymorphism analysis of highly virulent strains of infectious bursal disease viruses from Holland, Turkey, and Taiwan. Avian Dis. 1998; 42: 470-479. Ref.: https://goo.gl/X2QFdX
- Brown MD, Green P, Skinner MA. VP2 sequences of recent European ‘very virulent’ isolates of infectious bursal disease virus are closely related to each other but are distinct from those of ’classical’ strains. J Gen Virol 1994; 75: 675–680. Ref.: https://goo.gl/Qu2qxF
- Teshome M, Fentahunand T, Admassu B. Infectious bursal disease (Gumboro disease) in chickens. Br J Poult Sci 2015; 4: 22–28. Ref.: https://goo.gl/icaJr2
- Alkie TN, Rautenschlein S. Infectious bursal disease virus in poultry: Current status and future prospects. Vet Med Res Rep 2016; 7: 9-18. Ref.: https://goo.gl/S9iJrA
- Lukert PD, Saif YM. Infectious bursal disease. In: Saif Y. M. (Ed.) Diseases of Poultry (11th ed.). Iowa State University Press, Ames, Iowa. 2003; 161-179.
- Rosenberger JK, Cloud SS, Gelb J, Odor E, Dohms SE. Sentinel birds survey of Delmarva broiler flocks. Proc. of the 20th National Meeting on Poultry Health and Condemnation. Ocean City, MD, US. 1985; 94-101.
- Chettle N, Stuart JC, Wyeth PJ. Outbreak of virulent infectious bursal disease in East Anglia. Vet Rec 1989; 125: 271-272. Ref.: https://goo.gl/s15kYj
- Miller PJ, Estevez C, Yu Q, Suarez DL, King DJ. Comparison of viral shedding following vaccination with inactivated and live Newcastle disease vaccines formulated with wild-type and recombinant viruses. Avian Dis. 2009; 53: 39-49. Ref.: https://goo.gl/kNnjS2
- Khan MS, Maliga P. Fluorescent antibiotic resistance marker to track plastid transformation in higher plants. Nature Biotechnol.1999; 17: 910-915. Ref.: https://goo.gl/dqvyUL
- Khan MS, Hameed MW, Nozoi M, Shiina T. Disruption of the psbA gene by copy correction mechanism reveals that the expression of plastid-encoded genes is regulated by photosynthesis activity. J Plant Res. 2007; 120: 421-430. Ref.: https://goo.gl/eSjnDy
- Khan MS. Hybrid transcription-mediated transgene regulation in plastids. Trends in Biotechnol. 2006; 24: 479-482. Ref.: https://goo.gl/DeFDcv
- Bock R, Khan MS. Taming plastids for a green future. Trends in Biotechnol. 2004; 22: 311-318. Ref.: https://goo.gl/kKU3B3
- Suzuki K, Nakanishi H, Bower J, Yoder DW, Osteryoung KW, et al. Plastid chaperonin proteins Cpn60 alpha and Cpn60 beta are required for plastid division in Arabidopsis thaliana. BMC Plant Biol. 2009; 9: 38. Ref.: https://goo.gl/BhKFJe
- Daniell H, Khan MS, Allison L. Milestones in chloroplast genetic engineering: an environmentally friendly era in biotechnology. Trends in Plant Sci. 2002; 7: 84-91. Ref.: https://goo.gl/biyvjQ
- Khan MS. Utilizing heterologous promoters to express green fluorescent protein from jellyfish in tobacco chloroplasts. Pak J Bot. 2001; 33: 43-52. Ref.: https://goo.gl/oqLF5B
- Wu H, Singh NK, Locy RD, Gunn KS, Giambrone JJ. Immunization of Chickens with VP2 Protein of Infectious Bursal Disease Virus Expressed in Arabidopsis thaliana. Avian Dis. 2004; 48: 663 – 668. Ref.: https://goo.gl/S5EYPQ
- Wu J, Yu L, Li L, Hu J, Zhou J, Zhou X. Oral immunization with transgenic rice seeds expressing VP2 protein of infectious bursal disease virus induces protective immune responses in chickens. Plant Biotechnol J. 2007; 5: 570 – 578. Ref.: https://goo.gl/3REyng